Descripton of the OSSE Instrument
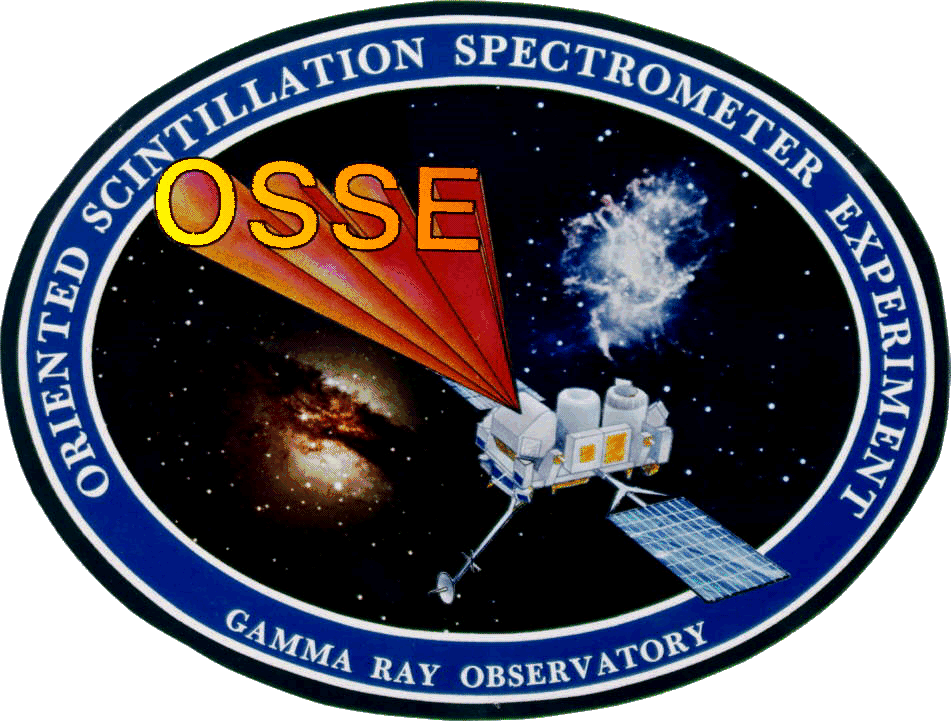
Click image for larger view
The Oriented Scintillation Spectrometer Experiment (OSSE) has been designed to undertake comprehensive observations of astrophysical sources in the 0.05-10 MeV energy range. Secondary capabilities for gamma-ray and neutron observations above 10 MeV have also been included, principally for solar flare studies. The use of NaI(Tl) scintillation techniques provides large area and high sensitivity in the 0.05-10 MeV region.
The highest sensitivity is maintained by implementation of an observational technique which minimizes susceptibility to background variations due to orbital geomagnetic latitude effects and spallation produced radioactivity. This technique uses an offset pointing capability to modulate a celestial source's contribution to the observed gamma-ray flux on a time scale short compared to the background variations, so that it can be reliably extracted from the background. Normally, OSSE performs two-minute viewings on and off the targeted source. Background variations are modulated by the spacecraft's orbital period of about 90 minutes.
The offset pointing capability also enables the experiment to undertake observations of selected sources (e.g., transient objects) with a significant independence of their location on the sky or on pointing restrictions which may be imposed by the spacecraft. For example, this capability will be used to monitor solar flare activity without impacting the planned observational program of the other Compton experiments. It will also be used to achieve a two-source per orbit capability wherein a secondary source will be studied during orbital periods of earth occultation of the primary source.
Four identical detector systems are used to obtain the operational flexibility to meet the variety of scientific objectives previously outlined. Each of OSSE's four detectors operates, to a large degree, as an independent experiment. They have independent electronic systems and pointing systems. The synchronization of the four detectors is provided by the central electronics, which provides the data acquisition timing and coordination of the pointing directions. As shown in Figure II-1, each of the detectors is mounted in a single axis orientation control system which provides offset pointing over a range of 192 in the spacecraft X-Z plane. The detectors are generally operated in co-axial pairs. While one detector of a pair is observing the source, the other detector can be offset to monitor the background. After a programmable time interval, typically two minutes, the detectors will interchange observation directions by opposite rotations. Each detector's maximum rotation (or slew) rate is 2 per second.
Figure II-2 displays the major components of one of the OSSE detectors. The primary element of each detector system is the NaI(Tl) portion of a 330-mm diameter NaI(Tl)-CsI(Na) phoswich consisting of a 102-mm thick NaI(Tl) crystal optically coupled to a 76-mm thick CsI(Na) crystal. Each phoswich is viewed from the CsI face by seven 89-mm diameter photomultiplier tubes (PMTs), providing an energy resolution of 8% at 0.661 MeV. Active gain stabilization is used to maintain this energy resolution by individually adjusting the gain of each of the seven PMTs (the absolute gain of the OSSE phoswich remains stable to within 0.1% during a typical two week observing period. Utilizing the differing scintillation decay time constants of NaI(Tl) and CsI(Na), the detector event processing electronics incorporates pulse-shape analysis for the discrimination of events occurring in the NaI crystal from those occurring in the CsI, allowing the CsI portion of the phoswich to act as anticoincidence shielding for the NaI portion. A tungsten alloy passive slat collimator, located directly above the NaI portion of each phoswich, defines the gamma-ray aperture of the phoswich detector, providing a 3.8 x 11.4FWHM rectangular field-of-view throughout the 0.1-10 MeV energy range. Each phoswich aperture is covered by a charged particle detector (CPD) consisting of a 508-mm square by 6-mm thick plastic scintillator which is viewed by four 51-mm diameter photomultiplier tubes and is used for charged particle background rejection (energy losses greater than a nominal threshold of 0.20 MeV in the CPD trigger rejection of coincident energy losses in the phoswich). Both the phoswich and tungsten collimator are enclosed in a 349-mm long by 85-mm thick annular shield of NaI(Tl) scintillation crystals which, together with the CsI(Na) portion of the phoswich and the CPD, forms the active anticoincidence shield for background rejection. The NaI(Tl) annular shield is divided into four optically-isolated quadrants or segments, each viewed by three 51-mm diameter photomultiplier tubes.
The tungsten collimators are installed in the detectors so that OSSE detector motion scans the source with the collimator's 3.8 FWHM field-of-view. A background offset of 4.5 provides optimum modulation of the source contribution. This background offset, however, is programmable so that alternate background offsets can be selected in source-confused regions or for other scientific reasons. the nominal observing sequence observes the background on both sides of the source target. the average of these two backgrounds will generally be used in the data analysis as the "background" for the source observation. This technique minimizes the systematic effects of modulation of gamma-ray background local to the spacecraft. A typical detector observing sequence might consist of continuous repetition of the following sequence:
- observe the source direction for 2 minutes
- move to a background position 4.5 degrees counter-clockwise from the source and observe for 2 minutes
- return to the source direction and observe for 2 minutes
- move to a background position 4.5 degrees clockwise from the source and observe for 2 minutes
In the 90 between the spacecraft Z and X axes, all four detectors can be used for observations. Outside this range, the upper pair and lower pair of detectors begin to intrude into each other's fields of view. In that situation, the upper pair of detectors can be programmed to view one source and the lower pair can view another, independent target.
The ability to select viewing directions which contain interesting targets well away from the spacecraft Z-axis allows OSSE to make good use of the Z-axis earth occultation periods; by selection of the spacecraft orientation at the start of a two week observation, OSSE can move its detectors between the primary Z-axis target and the secondary target each orbit without reorientation of the spacecraft.
The experiment's data acquisition and control system incorporates varied modes of operation depending on the type of information desired during a particular observation. Diagnostic capabilities and redundancy are important features of the system; the ability to re-configure the experiment in the event of a failure has also been included. This versatility is achieved through the use of redundant programmable microprocessors.
Energy losses (spectra) in the phoswich are processed by three separate pulse-height and pulse-shape analysis systems for each detector. A low range pulse-height analyzer (PHA) covers the energy range 0.05-1.5 MeV; a medium range PHA covers the energy range 1-10 MeV; and a high range PHA covers energies greater than 10 MeV, nominally 10-250 MeV. Pulse-shape discrimination in the highest range is also used to separate neutron and gamma-ray energy losses in the NaI portion of the phoswich by utilizing the differing time characteristics of the secondaries produced by these interactions.
Energy losses above 0.10 MeV in each of the four annular shield segments are detected and utilized for rejection of coincident energy losses in the phoswich. The energy losses in the 0.10-8 MeV range are also pulse height analyzed as part of a diagnostic calibration analysis system. The good spectral resolution of the shield segments (10.5% at 0.66 MeV) permit the use of shield spectra in the study of solar flares and possibly gamma-ray bursts. In addition, the low level discriminator event rates from the shields (set at 0.10 MeV, but programmable from 0.03 to 0.47 MeV) are processed by the OSSE central electronics for the detection and capture of gamma ray bursts. This gamma ray burst trigger operates independently from the trigger provided by the BATSE instrument.
The primary data from the OSSE instrument consist of time-averaged energy loss spectra from the individual detectors (spectral memory data). Each detector accumulates separate energy loss spectra from the validated gamma-ray events in each of the three phoswich energy ranges. The time interval for these accumulations, called the spectrum acquisition cycle time (TSAC), is a function of the overall operating mode of the OSSE experiment and is typically 16.384 or 32.768 seconds. The acquisition cycle time is controlled by the OSSE central electronics which selects the TSAC interval based on the OSSE telemetry format. Acquisition times in the range between 2.048 seconds and 32.768 seconds are available. The detectors' accumulation memories are double-buffered so that accumulation occurs in one memory while the other memory is being transmitted. The accumulation memories can support a maximum of 4096 events per spectral channel per TSAC.
Gamma-ray events for the two lowest phoswich energy ranges (0.05-1.5 MeV and 1-10 MeV) are separately accumulated into 256 channel spectra. These energy loss spectra have uniform channel widths of approximately 6 keV and 40 keV, respectively. the highest phoswich energy range (greater than 10 MeV) is processed to discriminate gamma-ray and neutron events. These gamma-ray and neutron events are then separately accumulated into 16 channel spectra. These energy loss spectra have uniform channel widths of approximately 16 MeV.
If high time resolution is required, e.g. for the analysis of fast pulsars, an alternate data mode is provided. In this mode (pulsar mode), spectral resolution or bandwidth is sacrificed in order to obtain time resolutions of up to 0.125 milliseconds. The pulsar mode processing permits the definition of up to eight energy bands to be included in the transmitted pulsar data. Gamma-ray events qualified as being in one of these eight energy bands are then processed in one of two pulsar modes:
- event-by-event mode, where selected events are time-tagged and both energy loss and arrival time of the event are transmitted in the telemetry, or,
- rate mode, where high time resolution rate samples are taken in each of the eight energy bands.
The pulsar rate mode can accommodate a much higher event rate but at the sacrifice of spectral resolution. This mode records the number of events in each of the defined energy bands at a specified sample frequency. The highest sample rate in this mode provides a resolution of 4 milliseconds. Sample times from 4 msec to 512 msec can be selected.
OSSE includes a gamma ray burst capture capability (burst mode) which is based on the measurement of the gamma-ray event rates in the NaI annular shields. These event rates are sampled by the OSSE central electronics on a selectable time scale of from 4 msec to 32 msec. A gamma ray burst trigger signal, either from BATSE or from internal processing, activates the storage of the next 4096 samples in a burst memory. A selectable fraction of this memory preserves the rate samples prior to the trigger. With coarser time resolution (approximately 1 second), rate samples from the individual NaI shield quadrants are available which can be used to identify the direction of the gamma ray burst. Selected shield spectra can also be accumulated using an on-board calibration and diagnostic PHA (CALPHA) in each detector subsystem.
The OSSE internal burst trigger monitors the annular shield rate samples for successive samples above a specified event rate as the indication of a gamma ray burst. The BATSE Burst Trigger Signal will be the primary burst trigger signal for OSSE since it incorporates more sophisticated trigger detection and a burst direction measurement which will be able to identify possible solar flares. The ability to react to solar flares will significantly enhance the OSSE science.
The OSSE instrument also carries a separate charged particle monitor (CPM) detector (see Figure II-1). This 19-mm diameter plastic scintillation detector is enclosed in a passive shield of approximately 3 g/cm^2 of aluminum and is viewed by a 19-mm PMT. The event rates in this detector provide a monitor of the high energy charged particle environment for OSSE. Additionally, it provides a detection of the spacecraft entry into the South Atlantic Anomaly (SAA). The spacecraft is designed to turn off the OSSE detectors during traversals of the SAA; this charged particle monitor, however, remains on to provide integral charged particle dose monitoring for background modeling.
If you have a question about CGRO, please contact us via the Feedback form.