CVs and supersoft sources
Cataclysmic variables (CVs) are a large, diverse class of semi-detached mass-exchanging binaries consisting of a white dwarf primary and a late-type (G, K, or M) secondary. In non-magnetic CVs, the material lost by the secondary forms a disk around the white dwarf and accretion onto the compact star occurs in a highly sheared boundary layer between the disk and the surface of the white dwarf. In the synchronously rotating AM Her stars or polars, the strong magnetic field (B ~ 10-100 MG) of the primary envelops the secondary, and the material lost by the secondary is funneled along the magnetic field lines and falls radially onto a small spot in the vicinity of the magnetic poles. In the standard picture, the accreting material passes through a standoff shock far enough above the surface of the white dwarf for the hot, post-shock material to cool and come to rest at the stellar surface. In the asynchronously rotating DQ Her stars or intermediate polars, the weaker magnetic field (B~ 0.1-10 MG) of the primary and the larger size (longer period) of the binary allow a disk to form, but the center of this disk is disrupted at that radius where the magnetic field of the white dwarf is strong enough to dominate the flow. The accreting material then leaves the disk and flows along the magnetic field lines to accrete onto the white dwarf in a manner similar to AM Her stars.ASCA has contributed significantly to our understanding of each of these three classes of CVs via its unique combination of large collecting area (necessary for these relatively dim sources), wide bandpass (necessary for these relatively hard multi-temperature sources), and high spectral resolution (necessary to resolve the K-alpha emission lines of Mg, Si, S, Ar, and Fe).
An excellent example of ASCA's spectroscopic capabilities is afforded by observations of non-magnetic CVs. Acceptable fits to ROSAT PSPC data can be obtained with either two-temperature thermal plasma spectra or single-temperature thermal bremsstrahlung spectra with a narrow emission line at ~ 1 keV (van Teeseling & Verbunt 1994 A&Ap 292, 519; Richman 1996 ApJ 462, 404). With their wide bandpass and high spectral resolution, the ASCA SIS and GIS spectrometers have demonstrated conclusively that the hard X-ray spectrum of the boundary layer emission of non-magnetic CVs is characterized by a two-temperature thermal plasma with kT_1 ~ 1 keV and kT_2 ~ 3-10 keV (Nousek et al. 1994 ApJ 436, L19; Watson & Osborne 1996 AAS/HEAD meeting). In addition to the emission lines of this high-temperature thermal plasma, the 6.4 keV Fe K emission line has been detected for the first time (Kitamura et al. 1996 Waseda meeting; Done & Osborne 1996 Waseda meeting; Watson & Osborne 1996 AAS/HEAD meeting). If this line is due to fluorescence, as seems likely, it implies that the hot gas is in close proximity to relatively cold photoionized material such as the surface of the white dwarf and/or accretion disk. That the hard X-rays come from a compact region near the white dwarf is demonstrated most convincingly by ASCA observations of the eclipsing dwarf nova HT Cas (Figure 22: Mukai et al. 1996 ApJ submitted). The factor of ~ 10 higher count rate for the ASCA SIS+GIS light curve relative to that of the ROSAT HRI is crucial in measuring the eclipse depth and width and hence in constraining the geometry of the X-ray emitting gas: it is found that the X-ray eclipse is deep, compatible with being total, and that the eclipse transition is short, placing a limit of 1.15 times the radius of the white dwarf as the total size of the X-ray emission region.
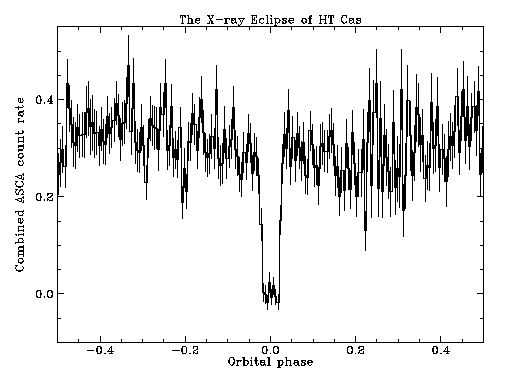
Figure 22. The combined ASCA SIS+GIS light curve of the dwarf nova HT Cas, a deep X-ray eclipse is obvious at orbital phase 0.0 (i.e., the phase of the optical eclipse of the white dwarf).
ASCA's ability to resolve the K-alpha emission lines of cosmically abundant elements has for the first time allowed measurement of the physical conditions in the post-shock region of magnetic CVs. The simultaneous presence of H- and He-like K-alpha emission lines of Mg, Si, S, Ar, and Fe in the ASCA SIS spectrum of the intermediate polar EX Hya (figure 23) clearly signals the presence in this system of a range of temperatures extending from ~ 0.9 keV to ~ 8 keV (Fujimoto & Ishida 1996 ApJ submitted). Via a model of the settling flow below the shock, the intensity ratios of the H- and He-like K-alpha emission lines constrain the shock and base temperature of the accretion column to kT_s = 15 +/- 2 keV and kT_b = 0.8 +/- 0.2 keV, respectively. This measurement for the shock temperature confirms the conclusion from Ginga data that the hard X-ray continua of magnetic CVs are the superposition of a kT_s ~ 15 keV thermal spectrum and its harder reflection spectrum from the surface of the white dwarf (Beardmore et al. 1995 MNRAS 272, 749; Done, Osborne & Beardmore 1995 MNRAS 276, 483). In addition, the shock temperature kT_s = 3GM_wd mu m_H/8R_wd is a function of M_wd/R_wd and R_wd is a function of M_wd via the mass-radius relation, so a very good estimate of the white dwarf mass is provided by ASCA spectroscopic measurements. For EX Hya, M_wd = 0.47 +/- 0.04 Solar masses , a result which is independent of the usual uncertainties involving K corrections and the inclination of the binary.
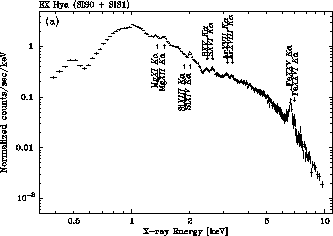
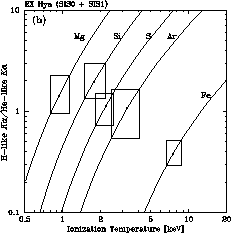
Figure 23. (a) ASCA SIS spectrum of the intermediate polar EX Hya showing the H- and He-like K-alpha lines of Mg, Si, S, Ar, and Fe. (b) Allowed range of ionization temperature for the observed line intensity ratio of each element.
ASCA has contributed significantly to our understanding of the continua of magnetic CVs as well. ASCA SIS observations of FO Aqr demonstrate the complexity of low-energy continuum of this intermediate polar (Mukai, Ishida, & Osborne 1994 PASJ 46, L87). The spectrum from 0.5 to 10 keV can be fit by a single kT ~ 30 keV thermal bremsstrahlung spectrum suffering varying amounts of absorption. Below ~ 1.5 keV, an unabsorbed component dominates, and above that energy the continuum is photoelectically absorbed by a range of column densities between N_H ~ 3 x 10^22 and 3 x 10^23 atoms/cm^2. That the unabsorbed and absorbed components originate in different regions is indicated by the fact that the strength of the beat period between the spin period of the white dwarf and the orbital period of the binary is a strong function of energy, with little to no beat modulation above ~ 3 keV. The continua of polars are similarly complex. It is known from Ginga observations that the hard X-ray continuum of AM Her can be fit by a thermal bremsstrahlung spectrum with kT ~ 14 keV with a partial covering absorber and reflection from the surface of the white dwarf (Beardmore et al. 1995 MNRAS 272, 749), but the model of the continuum in the ~ 2-5 keV bandpass is not unique. Similar to FO Aqr, the continuum of AM Her in the ASCA bandpass can be fit by a single temperature thermal bremsstrahlung spectrum suffering photoelectric absorption by a range of column densities between N_H ~ 9 x 10^19 and 2 x 10^23 atoms/cm^2 (see figure 24). Below ~ 0.7 keV, a soft component is detected which can be fit by a blackbody spectrum with kT = 36 +/- 3 keV. The peak of this soft component is detected in simultaneous EUVE observations, where it is observed that the blackbody is modified by the bound-free opacity of Ne VI (Paerels et al. 1996 ApJ in press).
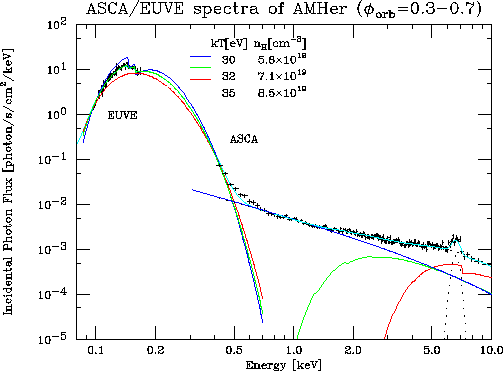
Figure 24. ASCA SIS and EUVE SW spectrum of the polar AM Her during magnetic phases phi = 0.3-0.7. The models are a single temperature thermals brems spectrum with three discrete values of N_H plus three blackbody spectra modified by interstellar absorption and the bound-free opacity of Ne VI.
Dozens of Super Soft Sources (SSS) have been discovered with Einstein and ROSAT (for reviews see e.g., Hasinger 1994 AIP Conference Proceedings 308, 611; and in Compact Stars in Binaries Cowley et al., Rappaport & Di Stefano, and Kahabka & Trumper). Steady nuclear burning on the surfaces of accreting white dwarves is a likely model for SSS (van den Heuvel et al. 1992 A&Ap 262, 97; Heise et al. 1994 A&Ap 288, L45).
Thus far, observational information on SSS in the X-ray band has been limited by the spectral resolution of the instruments used. While the ROSAT PSPC has a very suitable energy band (0.1-2 keV) for the study of SSS, its energy resolution (Delta E/E ~ 60 % at 0.5 keV) does not allow detection of spectral structures such as emission lines or absorption edges.
Having a superior energy resolution (Delta E/E ~ 10 % at 0.5 keV), the ASCA Solid State Spectrometer (SIS) is potentially a powerful instrument for the spectroscopic study of SSS, though its energy band (0.4-10 keV) is higher than the typical SSS energy range. RX J0925.7-475 is an unusual SSS having most of its X-ray emission above 0.5 keV (Motch et al. 1994 A&Ap 284, 827), and is thus the most suitable target for the ASCA SIS.
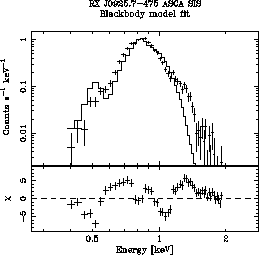
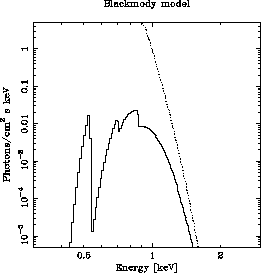
Figure 25. Left: Blackbody model fit result. Right: the best-fit model with (solid-line) and without (dotted-line) the interstellar absorption.
RXJ0925.7-475 was observed in the ASCA AO3 phase for 20 ksec. The ROSAT spectrum of RX J0925.7-475 is fitted with the blackbody having kT = 40-55 eV with an inter-stellar absorption of N_H = (1.0-1.9) x 10^22 atoms/cm^2 (Motch et al. 1994 A&Ap 284, 827). Figure 25 is a blackbody model fit to the ASCA spectrum, giving parameters close to the ROSAT best-fit ones. It is obvious that the fit is not acceptable (reduced Chi^2 ~ 10), and several absorption edge-like features are seen.
If the ASCA spectrum is fitted with with a blackbody model with absorption edges, at least three absorption edges are required at around 0.94 keV, 1.04 keV and 1.43 keV (figure 26). Introducing the absorption edges, the blackbody temperature significantly increases to 96 +/- 11 eV, and the bolometric luminosity and radius decreases, compared to the fit without absorption edges. This implies that SSS spectral parameters based on blackbody fits to Einstein or ROSAT spectra are subject to large uncertainties.
The ASCA spectrum allows a precise comparison of the observation and theoretical SSS spectral models. Ionization balance of hot white-dwarf atmospheres is determined by the surface gravity and the effective temperature, both of which can be constrained from model fitting to the data. The normalization of the model is directly related to the white-dwarf radius and the distance to the source. The radius, as well as the surface gravity, is almost uniquely determined using the white-dwarf mass-radius relation (e.g. Pringle and Webbink 1975 MNRAS 172, 493). Hence, ASCA observations of SSS enable us to determine the white-dwarf parameters, as well as the distance to the source.
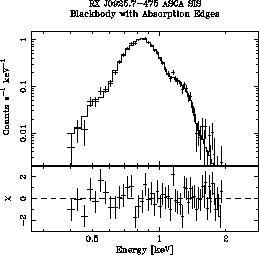
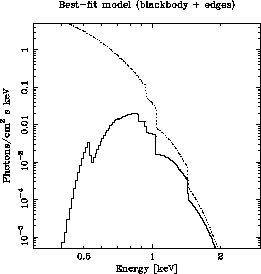
Last modified: Tuesday, 26-Jun-2001 14:22:33 EDT
If you have any questions concerning ASCA, visit our Feedback form.